Graphene Oxide
Graphite oxide sheets, now called graphene oxide (GO), are typically made from chemical exfoliation of graphite by reactions that have been known for 150 years. Interest in this old material has resurged since 2004 as it is considered a promising solution-processable precursor for bulk production of graphene.
We first entered this research area with a curiosity-driven hypothesis to study GO as an unconventional type of soft material, such as a 2D polymer, highly anisotropic colloid, membrane, liquid crystal, and amphiphile. We were driven by both this curiosity and the material processing challenges associated with its production and applications.
1. On the synthesis and processing of GO
2. High-throughput, high contrast imaging of GO and graphene-based sheets by FQM
3. New properties of GO sheets: Amphiphilicity
4. New properties of GO thin films: 2D nanofluidics
5. New properties of GO thin films: Molecular plasticity
6. Aggregation-resistant, self-dispersed crumpled graphene balls
1. On the synthesis and processing of GO
1.1 Fire risk of GO: The puzzle, methods of mitigation and opportunities
In many old literatures, GO solid was described as a flammable material that can combust upon heating. However, in recent literature GO and its graphene product have been demonstrated as flame retardant. We discovered that well purified GO, although can be easily reduced by heating, is quite stable against combustion even in a natural gas flame. However, when GO is contaminated by the alkaline salt byproducts generated in the synthesis, it becomes highly flammable due to salt catalyzed carbon combustion. The thermal instability of GO also inspired us to develop new material synthesis methods. For example, GO can be used as an in-situ power source to drive domino-like reactions to synthesize nanoparticle decorated graphene.
Also see:
-
C&E News: Graphite oxide’s flammability explained
-
Materials Views: Burning up
-
Chemistry & Industry: Fire risk for graphene oxide
-
Journal of Materials Chemistry: Chemically modified graphene: flame retardant or fuel for combustion?
1.2 Improved purification of GO
Purification of GO (e.g., by filtration, dialysis, centrifugation or freeze-drying) has been quite tedious and challenging due to its tendency to gel. To mitigate the fire risk of GO, especially during large scale production, we developed a procedure to suppress its gelation during washing and thus greatly increased the throughput of sample purification. When applied to filtration, this can significantly reduce processing time.
Improved 2-step washing of GO
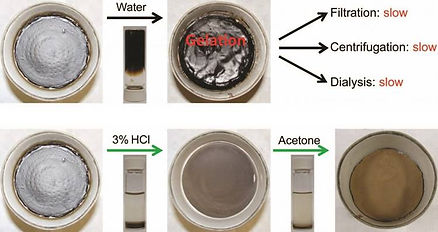
1.3 Photothermal reduction and patterning of GO films
We found that photothermal reduction and patterning of GO films and their polymer composites. This can be done using the Xenon flash lamp on a camera, which has sufficient energy to trigger the exothermic deoxygenation reaction of GO, and photothermally heat up the reduced GO to weld it with polymer particles.
Reduction and patterning of graphite oxide by camera flash
Also see:
-
C&E News: Chemical year in review 2009
-
C&E News: Making graphene in a flash
-
Current Science – Research News: A ‘FLASH’ in the synthesis of graphene
-
1.4 Domain-selected etching of GO and porous GO sheets
Oxidative etching of graphene flakes was observed to initiate from edges and the occasional defect sites in the basal plane, leading to reduced lateral size and a small number of etch pits. In contrast, etching of highly defective graphene oxide and its reduced form resulted in rapid homogeneous fracturing of the sheets into smaller pieces. On the basis of these observations, a slow and more controllable etching route was designed to produce nanoporous sheets by hydrothermal steaming. These porous sheets provide new opportunity for constructing bulk GO structures.
Steam-etched porous GO sheets
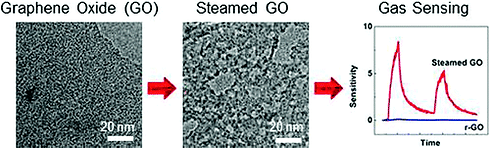
1.5 On the stability of GO films in water and their mechanical properties
GO films have long been known to be highly stable in water and this property has made their recent uses in membrane applications in solution possible. However, this is counterintuitive because GO sheets become negatively charged on hydration and the membrane should disintegrate owing to electrostatic repulsion. We discovered a long-overlooked reason behind this apparent contradiction. Our findings show that neat GO membranes do, indeed, readily disintegrate in water, but the films become stable if they are crosslinked by multivalent cationic metal contaminants. Such metal contaminants can be introduced unintentionally during the synthesis and processing of GO, most notably on filtration with anodized aluminum oxide (AAO) filter discs that corrode to release significant amounts of Al3+.
This finally solves the puzzle of the stability of GO films in water. This finding also helps to better understand the mechanical properties of GO thin films and some other puzzles about ionic cross-linking of GO films.
Graphene Oxide Membrane in Water To dissolve or not to dissolve
Also see:
-
C&E News: Why graphene oxide thin films are water stable
-
Science – Editor’s choice: For stability just add some debris
-
Materials Today: Graphene oxide stability
-
Materials 360: Mystery of graphene oxide membrane’s stability in water solved
-
Women in Nanoscience: Uncovering the mystery of graphene oxide paper (featuring Che-Ning Yeh)
2. High-throughput, high contrast imaging of GO and graphene-based sheets by FQM (click here to see more)
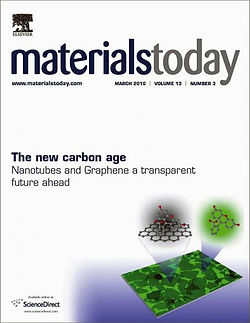
3. New properties of GO sheets: Amphiphilicity
3.1 GO as 2D surfactant sheets and interfacial assembly
We hypothesized that GO could act like sheet-like surfactant with interfacial activities, and verified that they indeed tend to migrate to interfaces (e.g., air-water, oil-water, and water-solid interfaces) and lower interfacial energy. This insight has led to better understanding of the solution properties of GO, and a new application of GO as a colloidal surfactant for dispersing insoluble materials such as carbon nanotubes. For example, like a molecular surfactant, GO can be directly spread on water surface and controllably assembled into monolayers by Langmuir-Blodgett technique.
It was also discovered that the amphiphilicity of GO is dependent on its size and pH, which allows the microstructures (e.g., wrinkles and overlaps) of such GO monolayers to be tuned to optimize the optoelectrical properties of the resulting graphene monolayers. Through collaboration, we also showed that interfacial assembly of GO sheets can create windows of liquid cells to achieve environmental X-ray photoelectron spectroscopy (XPS) and scanning electron microscopy (SEM).
Also see:
-
Nature Nanotechnology: Electron spectroscopy: A new window opens
-
Industrial Materials: 舊材料的新見解-氧化石墨烯之界面活性
-
Nature Chemistry – Research Highlights: Graphene oxide: Surfactant sheets
-
Nanowerk-Spotlight: New findings about the activity of graphene oxide sheets at interfaces
-
Ars Technica: Graphene oxide, world’s thinnest bar of soap
-
BE Etats-Uni: Le graphène renforce son statut de matériau star (In French)
-
C&E News: Carbon As Thin As Can Be
-
JACS image challenge: Test your knowledge of colloidal stability
-
JACS Podcast: An interview with Prof. Huang about lilypad-like assembly of GO

3.2 GO nanocolloids
The size-dependent amphiphilicity of GO motivated us to synthesized its nanocolloids by chemically exfoliating commercial graphite nanofibers, in which the graphene sheets are coin-stacked along the fiber length. In this way, the upper size limit of the resulting GO nanocolloids (c.a. tens of nanometers) is predetermined by the fiber diameters, leading to much more uniform and controllable later dimensions than fracturing-based size-reduction process. The resulting GO nanocolloids indeed have much improved colloidal stability in aqueous solutions, even when reduced to its graphene form. GO nanocolloids were found to have extraordinary performances in biosensing by us (in collaboration with Prof. Dravid’s group) and later many others.
Also see:
-
Materials Today: GO nano
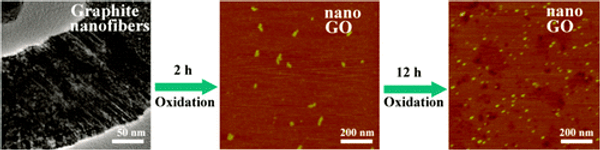
3.3 All-carbon composites for photovoltaics
GO can be used as surfactant to disperse other insoluble materials, especially π-conjugated systems such as carbon nanotubes in water. Various types of water-processable all carbon composites can be created using GO as surfactant. GO/SWCNTs thin films can replace the corrosive conducting polymer based interfacial layers used in organic solar cells for both single layer and tandem architectures. In another example, an all-carbon composite made of fullerene, co-dispersed with SWCNTs in water by GO, was found to yield photovoltaic effect. This leads to the hypothesis of all-carbon solar cells.
Towards all-carbon solar cells
Also see:
-
C&E News: Researchers produce an all-carbon composite solar cell from organic components suspended in water
-
Renewables International: New recipe for photovoltaics: a pinch of buckyballs, a dash of nanotubes, a sprinkling of graphene. Just add water and mix!
-
Fast Company Magazine: Making Solar Panels As Ubiquitous And Efficient As Leaves
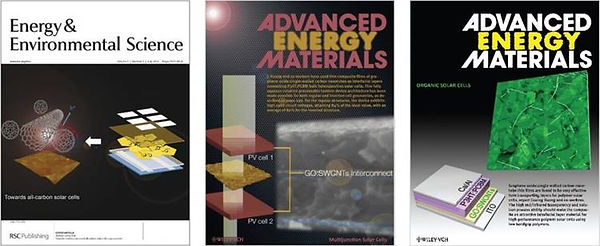
3.4 GO as a catalytic surfactant for carbonization reactions
GO was found to act as a catalytic surfactant to promote the hydrothermal carbonization reaction of carbon hydrates. In the presence of minute amount of GO (e.g., 0.1 wt%), carbonization proceeds much faster and yields more conductive carbon materials with high electrochemical specific capacitance comparable to graphene-based materials themselves. This demonstrates the potential of using catalytic amount of GO to significantly enhance the properties of other materials.
GO catalyzed biomass carbonization

4. New properties of GO thin films: 2D nanofluidics
In this line of work, we introduced a new concept bridging the fields of layered materials and nanofluidics. We demonstrated that the empty space between stacked GO sheets (and other charged 2D sheets) can be employed as 2D nanofluidic channels to enhance the ionic conductivity of electrolytes. This provides a materials-based alternative to expensive lithography-defined nanofluidic systems. Click here for more.
Nanofluidic ionic transport between GO sheets
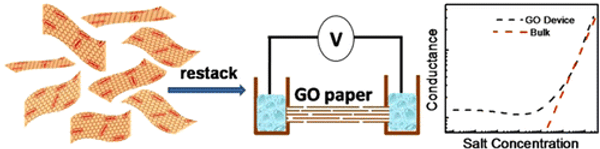
5. New properties of GO thin films: Molecular plasticity
In a collaboration with Profs. SonBinh Nguyen and Horacio Espinosa, a new bond-selective chemical transformation was discovered in GO membranes. Mechanical stretching of GO single layers was found to trigger epoxide-to-ether transformation, which deviates from the typical epoxide ring-opening reactions. This mechanochemical transformation confers pronounced plasticity and damage tolerance to GO monolayers.
Molecular plasticity in graphene oxide
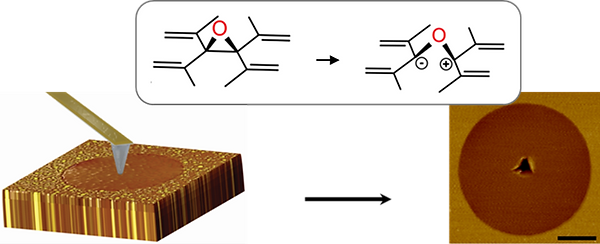
6. Aggregation-resistant, self-dispersed crumpled graphene balls
A major problem in the manufacturing and applications of bulk graphene materials is their tendency to aggregate due to strong van der Waals attraction. Restacking of sheets not only reduces their processability, but also compromises their properties such as accessible surface area. To address this challenge, we hypothesized and then demonstrated crumpled graphene balls, which have a unique shape that prevents tight packing, leading to weak inter-particle attraction. Click here to find out more.